Newsletter 3/2022
Our experts Dr. Kay-Uwe Giering and Andy Heinig shed light on how to set up a quantum system interface in the outside world.
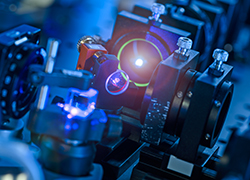
The transition of the quantum mechanics realm into engineering applications is opening up a large number of disruptive quantum technological opportunities. Their success relies on the recent technological advancements, which enable the controlled creation of individual quantum mechanical systems as well as their direct manipulation and measurement. Quantum technological systems purposefully exploit principles of quantum mechanics that are not encountered in everyday experience. For example, a quantum system can exist in two or more different states simultaneously, with the indeterminacy only being removed by a measurement (superposition principle). In this way, quantum mechanics furthermore allows a strong non-local correlation (entanglement) of system components, which is quintessential for many applications.
Quantum technologies encompass three subfields: Quantum computing exploits the superposition and further principles to parallelize computations. As a result, quantum computers will be able to solve optimization tasks and simulations in the domain of pharmacy, chemistry, materials science, or genetics, and many other areas that are unattainable with current conventional computers (quantum supremacy). For example, a quantum algorithm can solve the prime factorization task almost exponentially faster than the best-known conventional algorithm. However, since the security of many current encryption methods (such as RSA key exchange) relies on the classical algorithmic complexity, quantum computing renders current communication schemes (telephony, email, file transfer, instant messaging, online banking via https, etc.) vulnerable. Therefore, quantum communication is developing methods of key exchange that are unconditionally secure against unauthorized access and that are based on quantum principles. Finally, quantum sensing allows high-precision measurements of, for example, magnetic, electric, or gravitational fields, and has applications in areas such as microscopy, medicine, time measurement, and geophysics. While quantum sensing systems that beat the precision of their classical counterparts are already available commercially, the quantum computing and communication branches are presently developing prototypic systems at an early stage of commercialization
Microelectronics plays a crucial role in harnessing quantum technologies as future key technologies. On the one hand, semiconductor processes are an important part of creating quantum technological systems. Above all, however, high-performance electronic chips are needed to control the quantum setups and process the resulting extensive measurement data. Microelectronics thus provides the interface from quantum systems to the outside world. In addition to the performance requirements, some applications require that the systems are cooled to extremely low temperatures. This results in additional requirements for the mechanical structure and for the electrical design of the circuits.
They require high performance and frequent customization. At the same time, they are produced in small quantities. These conflicting demands pose a major challenge for the design and manufacture of the electronics. Compared to other applications, the quantities will not be particularly large even if quantum applications reach a commercial breakthrough point. On the other hand, many quantum applications often require highly customized circuits, for example, in terms of the voltage levels they need to process or provide. Furthermore, the data processing requirements are sometimes extremely high, so that only the most modern circuit concepts and circuits can meet them. Often, the electronics must also be fit in the smallest possible installation space, either due to the requirements of the application or because it is located in a cryostatic domain. Therefore, novel design concepts such as chiplets are expected to meet these requirements.
Chiplets make it possible to realize data processing with standard or special processors. Moreover, fast analog-to-digital converters (ADCs) or digital-to-analog converters (DACs) will be available as chiplets. With these converters, it would only be necessary to individually design the components that need to be specially adapted. For example, drivers as chiplets could provide the necessary voltage levels. Special technologies such as silicon-germanium semiconductor technology (SiGe) can also be used for this purpose and integrated as chiplets.
Chiplet technology thus offers a way to meet the various requirements for performance and customization in small quantities – while retaining a moderate cost level.